In this blog, we describe the mechanisms of action of the Clostridium difficile A and B toxins, and discuss their use in research and medicine. The Native Antigen Company provides biologically active C. diff toxins, as well as inactivated toxoids for a range of research applications.
The Clinical Burden of Clostridium difficile
Clostridium difficile (originally Bacillus difficilis) was first characterised in the 1930s from the gut microbiota of a healthy infant using Gram stain and light microscopy [1]. The subsequent name, C. difficile reflected the difficulty in isolating and culturing the pathogen in anaerobic environments. This new bacterial species was largely overlooked by the medical community in the following decades, with occasional reports suggesting an association with enteric disease. However, in 1978, a series of studies began to identify C. diff as the primary causative agent of pseudomembranous colitis (PMC) – A disease characterised by inflammation of colon’s epithelial layer [2, 3, 4, 5]. Patients that presented with C. diff infection were often on a course of clindamycin antibiotics, which spurred further investigations that collectively showed strong correlations between C. diff infection, PMC and antibiotic therapy [6, 7, 8]. Like most enteric bacteria, C. diff causes a wide spectrum of disease, ranging from mild mucosal inflammation and diarrhoea, to severe necrosis of gut tissue [9]. The Clostridia class of bacteria are an ancient lineage that are estimated to have diverged from the bacterial domain around 2.3 billion years ago as atmospheric oxygens levels were rising [10]. Due to advances in NGS, the Clostridia class has undergone recent taxonomic revisions and the is now formally known as Clostridoides, though the Clostridia name is still accepted [11].
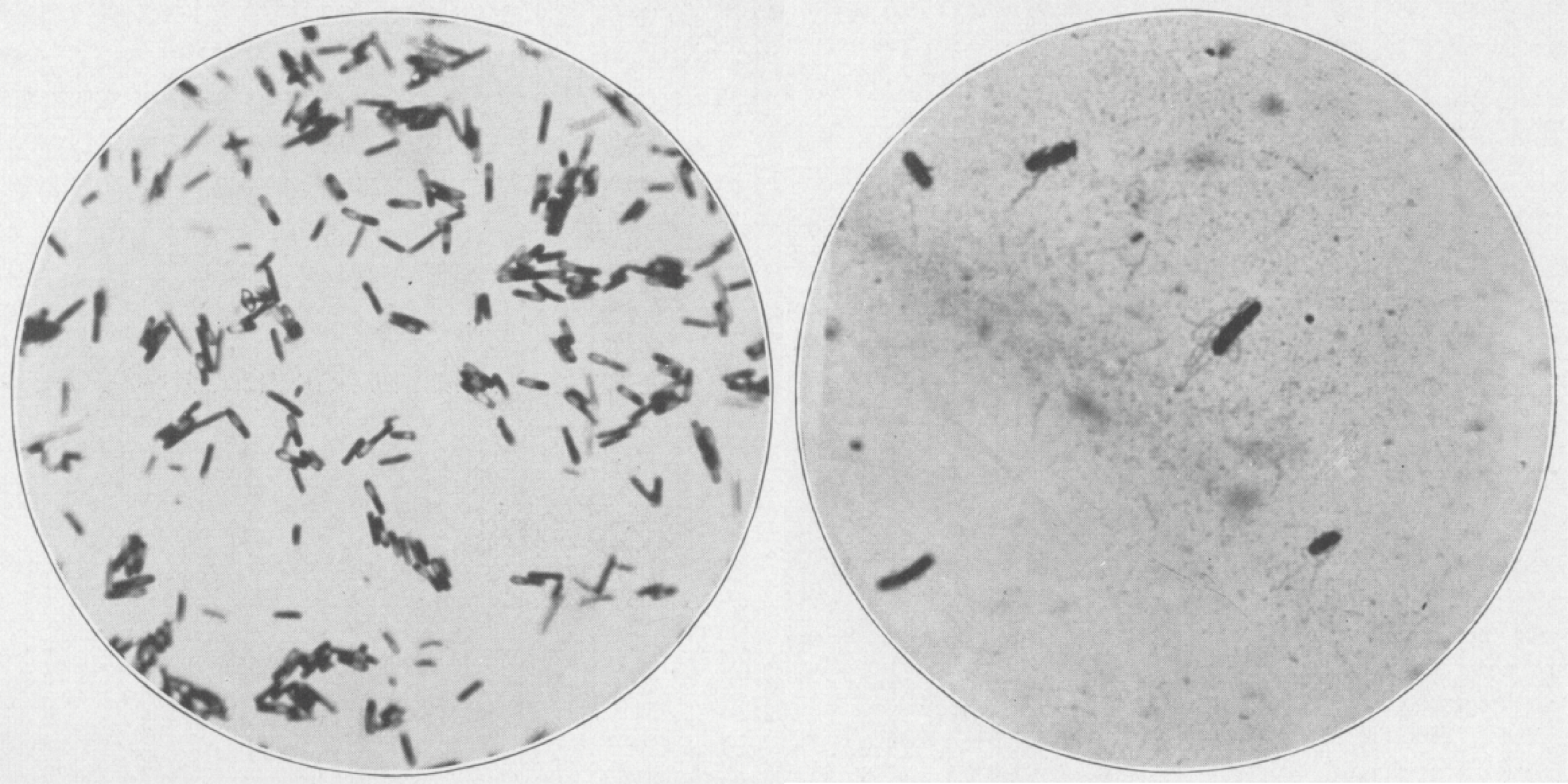
The first images of C. diff, shown by gram stain on blood agar, under X500 and X1,000 magnification, respectively [1].
Since its discovery, C. diff has emerged as a major cause of diarrhoea in the develop world. Its ability to spread readily in enclosed environments and its very hardy, resistant spores, have gained it notoriety as a significant cause of hospital-acquired infections. These infections are typically caused by exposure of intestinal microbiota to antibiotics that are not effective against C. diff, which disrupts the natural flora and allows commensal C. diff to flourish unchecked. In the United States alone, C. diff was estimated to cause half a million infections and 29,000 deaths in 2012 [12]. The economic burden is equally as substantial, with each C. diff infection estimated to result in over $3,600 of health care costs in the USA, costing over $1 billion annually [13]. Likewise, an estimated 125,000 C. diff cases are estimated to occur in Europe annually, causing 3,700 deaths [14].
Despite C. difficile’s clinical burden, we don’t have a very clear understanding of how it causes disease in humans, with a lot of active research still ongoing in this area. The nature of C. diff’s emergence is also unclear, though use of antibiotics and chemotherapeutics are thought to be significant drivers [15], causing many distinct C. diff strains to develop a high degree of multi-drug resistance and hypervirulence in recent years [16]. Interestingly, a recent study even linked the emergence of hypervirulent strains to an increased ability to metabolise ever-more present food additives [17].
Clostridium Toxins A and B
Despite a lack of understanding of the disease that C. difficile causes, there has been significant progress in the characterisation of its two major virulence factors, toxins A (TcdA) and B (TcdB) – glucosyltransferases that modify and inhibit the human Rho and Ras families of intracellular GTPases. TcdA/B are located on C. diff’s pathogenicity locus (PaLoc), along with the tcdR, E and C genes that carry out regulation and secretion [18].
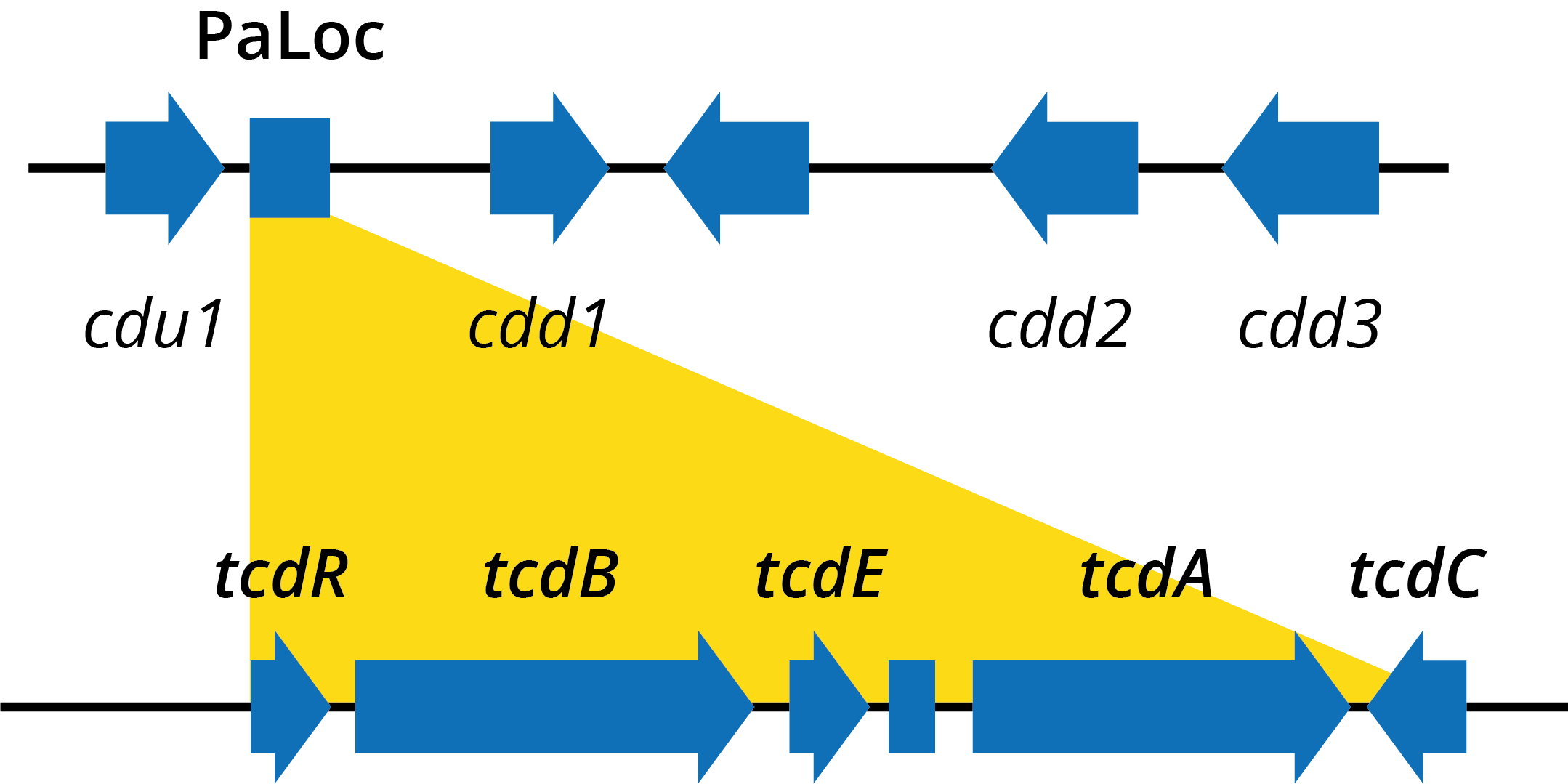
Diagram of genes within the Clostridium difficile pathogenicity locus.
TcdA and TcdB are 308kDa and 270kDa proteins, respectively, each consisting of four major functional domains: ABCD [19]. The N-terminal domain (domain A) is biologically active and contains the glucosyltransferase (GT) activity responsible for modifying target proteins; The C-terminal domain (domain B) is thought to be responsible for cell surface receptor recognition and binding; Domain C is an autoprotease (APD) domain that facilitates cleavage and the release of domain A; Domain D is likely involved in the delivery (TD) of the toxins from the endosome to the cytosol [19].
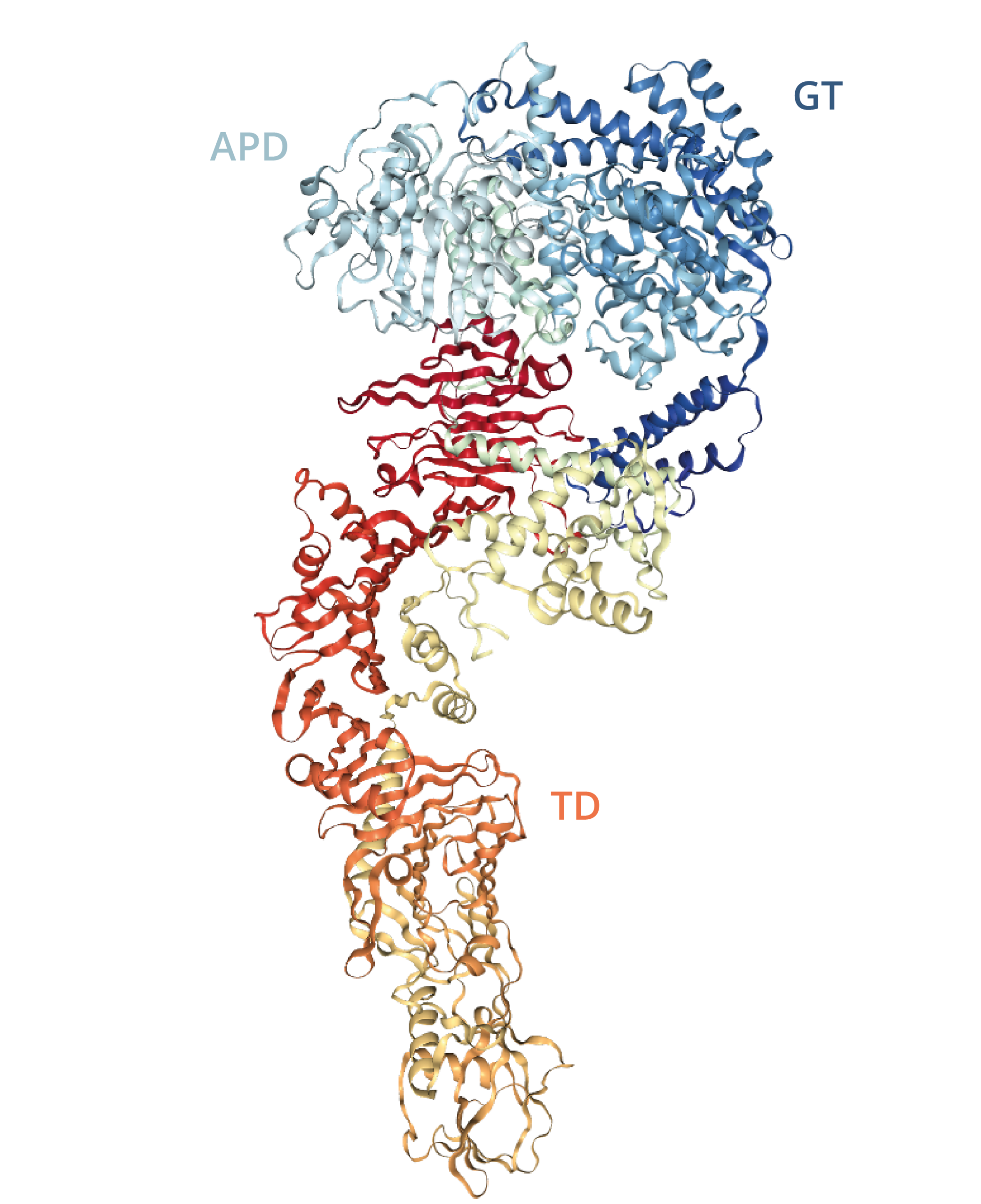
Diagram of TcdA autoprotease (APD), glucosyltransferase (GT) and toxin delivery (TD) domains [21].
The B domain (not shown above) at the toxin’s C-terminus is characterised by repetitive sequences known as combined repetitive oligopeptides (CROPs), which comprise a solenoid fold of 7 long, and 21 or 31 short repeats, followed by a hairpin loop [19]. CROPs are thought to mediate receptor recognition and binding. Both TcdA and TcdB share the solenoid motif, but differ in the spatial arrangements and number of repeat units, which is thought to affect their receptor-binding affinity [19, 21]. TcdA’s B domain binds surface carbohydrates and some cell-surface proteins, whereas TcdB binds a broad range of cell-surface proteins, including the Frizzled family [22]. However, it is worth noting that recent findings have suggested that the role of CROPs is less clear. CROPs do not bind all identified TcdA/B receptors and have only shown to bind weakly to carbohydrates in the case of TcdA, suggesting the presence of other receptor-binding structures [19]. This has led to speculation that CROPs may facilitate non-specific toxin-cell surface binding, to mediate more specific binding interactions [23].
Mechanisms of Action
The TcdA/B toxins are key to the pathogenesis of Clostridium difficile, and are responsible for mediating most of the symptoms of infection. The TcdA/B toxins achieve pathogenesis through a shared mechanism of action:
1) The series of events that leads to cell intoxication starts with toxin endocytosis by gut epithelial cells. Once TcdA/B binds a receptor, it is internalised and enters the cell via an endosome. TcdA and B appear to use different endocytotic pathways to achieve this, which is likely a result of their distinct receptors [19].
2) As the endosome acidifies, TcdA/B’s translocation (TD) domain elongates, preventing contact with CROPs, and inducing membrane insertion and formation of a pore [19].
3) TcdA/B’s structural rearrangement allows its autoprotease domain to cleave the N-terminal domain at a cysteine residue and release the glucosyltransferase domain into the host cell’s cytosol.
4) Once released and enzymatically active within the cell, the GT domain catalyses the transfer of a glucose molecule from UDP-Glucose to specific residues and inactivates the target protein. The inactivation of the Rho/Ras GTPases has a dramatic influence on cell physiology, ranging from cellular structure, to downstream signalling pathways.
In most cases, cells cannot withstand intoxication with TcdA/B, even at very low doses causing cell death. Widespread necrosis of tissue is thought to occur as a result of the reactive oxygen species (ROS) and reactive nitrogen species (RNS) released via apoptosis, which proliferate rapidly to neighbouring cells [24].
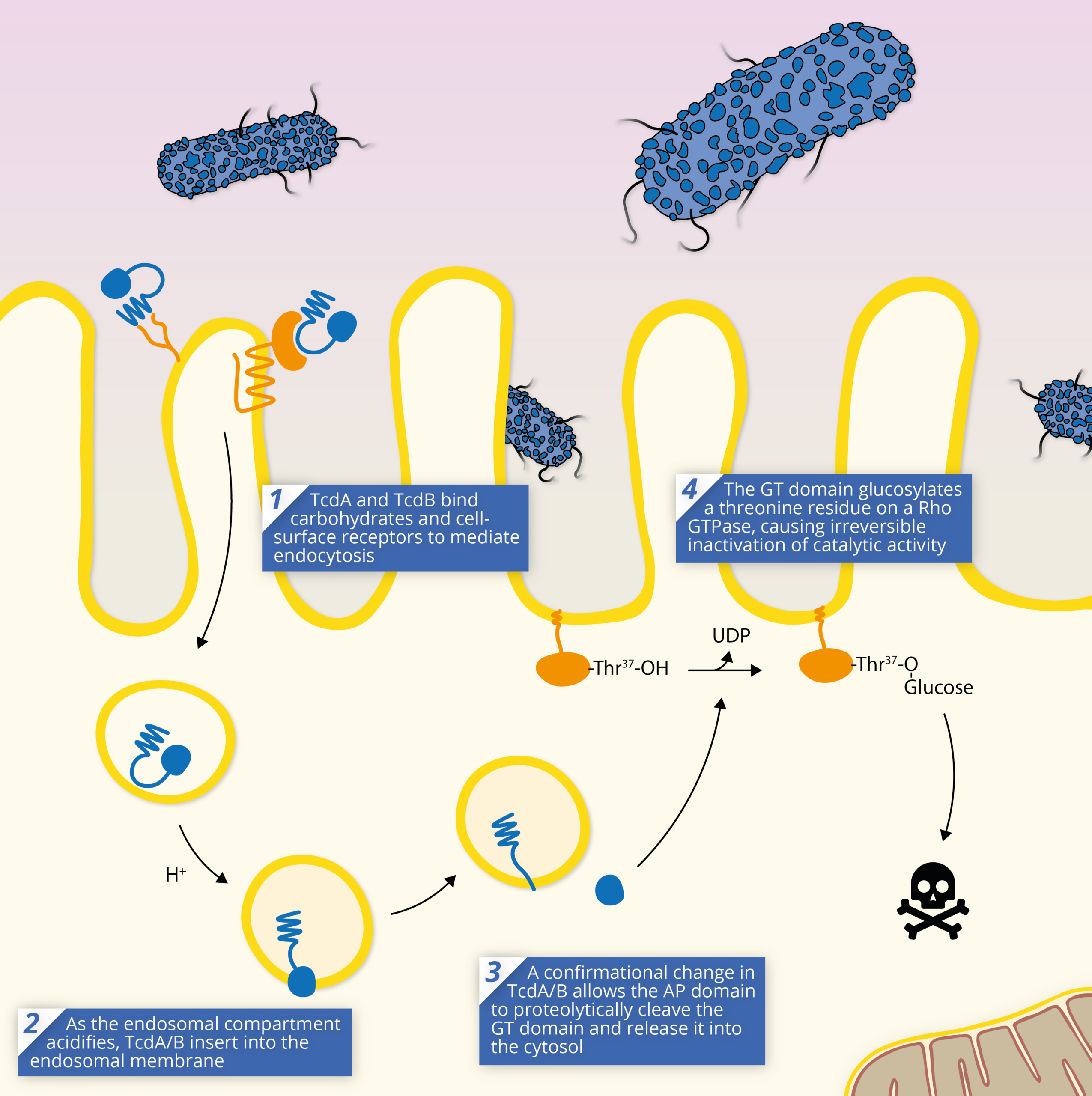
Medical Applications
By understanding the mechanisms of TcdA/B, it has possible to use them to investigate basic cellular biology. The ability of TcdA/B toxins to inactivate Rho, Rac and Cdc42 at sub-cytotoxic doses has facilitated the study of various cellular processes, including cell division, exocytosis, phagocytosis and cytoskeleton regulation [25]. In addition to use in fundamental research, clostridial toxins have also been investigated for human therapeutic applications. TcdA/B are efficient cell cytosol delivery vehicles and apoptosis-inducing compounds that make them potential candidates for therapeutic applications.
Antitoxins
One of the applications of TcdA/B has been in the development of a botulism antitoxin. By introducing two point mutations, it is possible to eliminate TcdA/B toxicity, without affecting endosomal uptake and translocation and specific TcdA/B domains can be switched out for structurally similar domains from other toxins, to modulate their effects [26]. A drawback of using a TcdA/B delivery system is its lack of cell specificity. In contrast, the botulinum toxins (BoNT) produced by the related botulinum species of Clostridia are known to show a high degree of neuronal cell specificity, inhibiting neurotransmission and causing flaccid paralysis. This occurs when BoNT’s endopeptidase domain inactivates the cytosolic proteins responsible for neurotransmitter release. While BoNT antagonists have been developed, their cytosolic delivery remains a challenge [27]. Therefore, a viable means of facilitating the delivery of such agents is through recombinant engineering with TcdA/B, which have evolved for this specific purpose.
A 2012 study engineered expression vectors for chimeric TcdB with an alkylguanine-DNA alkyltransferase domain (AGT-TcdB) for visualisation of localisation [28]. The domain was shown to retain some functionality and intoxicated Vero cells comparably to wild-type TcdB. The AGT-TcdB’s receptor-binding domain was then exchanged for its neuron-specific equivalent from BoNT, to generate the AGT-TcdB-BoNT/A-Hc chimera.
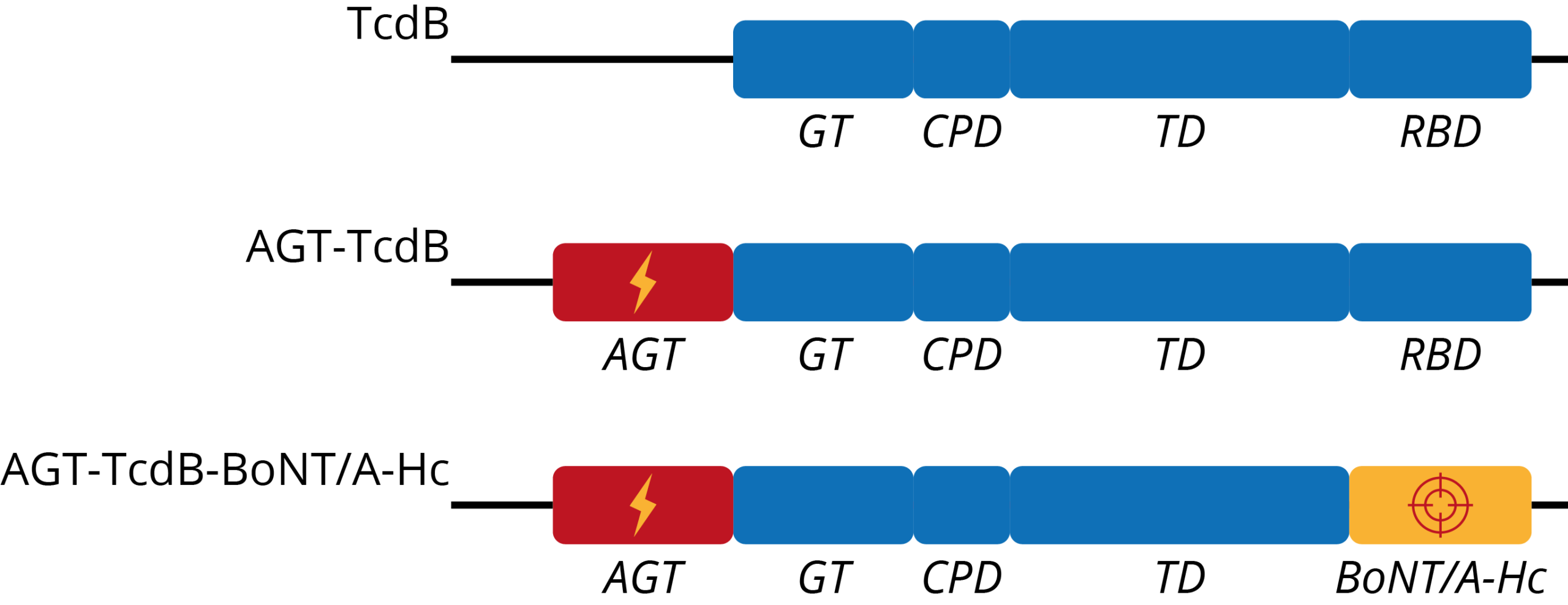
Diagram showing the gene structures of TcdB, an AGT-TcdB chimera, and an AGT-TcdB-BoNT/A-Hc chimera, developed as a botulinum antitoxin [28].
The addition of the BoNT receptor-binding domain showed a 25-fold increase in inducing toxic sensitivity in target cells, compared to AGT-TcdB, as well as an increased rate of intoxication. However, AGT-TcdB-BoNT/A-Hc did retain a degree of toxicity against non-neuronal cells – likely a result of additional receptor-binding regions found on TcdB [29]. This said, further characterisation and mutagenesis of key binding residues would likely ablate off-target effects, while retaining neuronal cell specificity. Therefore, not only did the results demonstrate the viability of producing BoNT antitoxins through a TcdA/B construct, but also imply that clostridial toxins are suitably flexible for a broad range of therapeutic applications.
Anticancers
TcdA/B’s ability to arrest the cell cycle and induce apoptosis has also made the TcdA/B toxins candidates for new cancer therapies. The cell death that these toxins induce is also highly immunogenic and has shown to induce potent host immune responses that could target MDR-tumours [30].
A study investigated the use of TcdB to inhibit breast cancer cell proliferation and stimulate anti-tumour specific immune responses [31]. When a breast cancer cell line was treated with varying concentrations of TcdB, it was shown to induce apoptosis, suppress proliferation and migration, and decrease the abundance of S-phase cells. TcdB also inhibited tumour growth in a mouse model and stimulated a strong inflammatory response. A following study investigated TcdA’s effects on a myelogenous leukaemia cell line, showing potent cell-cycle arrest and apoptosis [32]. TcdA’s ability to inhibit cell signalling pathways also suggested that it could enhance the inhibitory effect of other anti-cancers and partly reverse multi-drug resistance.
The application of C. diff toxins as cancer therapeutics is still very nascent, with further studies necessary to evaluate their practical clinical use. With the development of more complex fusion proteins that utilise specificity domains and on/off regulation, new means of treating tumours and MDR may be achieved.
Toxins, Toxoids and Antibodies from The Native Antigen Company
The Native Antigen Company offers a range of highly purified Clostridium difficile A and B toxins in a variety of ribotypes, including the pathogenic ribotypes, 027 and 078. These toxins can be used as positive controls in diagnostic assays, in the determination of assay range, cytotoxicity testing, or for basic research purposes. Our toxins are also available in range of volumes and are lyophilised for ease of use. In addition, we offer C. diff toxoids, which are derived from our native toxins and inactivated with formaldehyde.
To complement our range of C. diff toxins, we also offer monoclonal anti-TcdA and anti-TcdB mouse antibodies. These antibodies do not cross-react between toxin types and are suitable for use in immunoassay development and toxin detection.
References
1. https://jamanetwork.com/journals/jamapediatrics/article-abstract/1176814
2. https://www.thelancet.com/journals/lancet/article/PIIS0140-6736(78)90912-1/fulltext
3. https://www.ncbi.nlm.nih.gov/pmc/articles/PMC1603073/
4. https://www.ncbi.nlm.nih.gov/pmc/articles/PMC421886/
5. https://www.thelancet.com/journals/lancet/article/PIIS0140-6736(78)93001-5/fulltext
6. https://www.ncbi.nlm.nih.gov/pubmed/7300801
7. https://www.ncbi.nlm.nih.gov/pubmed/7053190
8. https://www.ncbi.nlm.nih.gov/pmc/articles/PMC493340/
9. https://www.ncbi.nlm.nih.gov/pubmed/18177220
10. https://www.tandfonline.com/doi/abs/10.1080/01490450303891
11. https://www.sciencedirect.com/science/article/abs/pii/S1075996416300762?via%3Dihub
12. https://www.ncbi.nlm.nih.gov/pubmed/25714160/
13. https://www.ncbi.nlm.nih.gov/pubmed/11774082/
14. https://www.ecdc.europa.eu/en/clostridium-difficile-infections/facts
15. https://www.ncbi.nlm.nih.gov/pmc/articles/PMC1082799/
16. https://jcm.asm.org/content/55/7/1998
17. https://www.nature.com/articles/nature25178
18. https://www.ncbi.nlm.nih.gov/pmc/articles/PMC4597214/
19. https://www.ncbi.nlm.nih.gov/pubmed/28657883
20. https://www.ncbi.nlm.nih.gov/pubmed/27512603
21. https://www.ncbi.nlm.nih.gov/pmc/articles/PMC3060812/
22. https://www.ncbi.nlm.nih.gov/pmc/articles/PMC5655507/
23. https://www.ncbi.nlm.nih.gov/pubmed/26602083
24. https://www.pnas.org/content/110/46/18674
25. https://www.ncbi.nlm.nih.gov/pmc/articles/PMC1082799/
26. https://www.ncbi.nlm.nih.gov/pmc/articles/PMC3434558/
27. https://journals.plos.org/plosone/article?id=10.1371/journal.pone.0020352
28. https://www.hindawi.com/journals/jt/2012/760142/
29. https://academic.oup.com/glycob/article/18/9/698/1988008
30. https://www.ncbi.nlm.nih.gov/pubmed/27271747
31. https://www.sciencedirect.com/science/article/pii/S0753332217360973?via%3Dihub
32. https://www.ncbi.nlm.nih.gov/pmc/articles/PMC5841023/