The development of a cytomegalovirus vaccine has been 50 years in the making. Are there any technologies in the pipeline that could prompt a breakthrough? This article was originally posted online at The Medicine Maker, a Texere publication.
To say that cytomegalovirus (CMV) is common would be an understatement. The virus, which belongs to the Herpesviridae family, infects the majority of people over the course of their lifetime, making it one of the most ubiquitous human pathogens in the world (1, 2). And yet general awareness of CMV and its impact on human health (particularly the risk of congenital infection) is relatively poor.
CMV has one of the largest viral genomes known to man and is composed of hundreds of proteins (see Figure 1). It exists as a variety of interrelated strains, both within the environment and those it infects (3).
Though we don’t yet have a full picture of how CMV is transmitted, the virus appears to spread via a range of human bodily fluids, including urine, saliva, blood and tears. Once inside the body, it invades a remarkably broad range of cell types to mold cellular functions that support its replication. Fortunately, the immune system typically responds quickly, and drives the virus into a latent state, where it remains dormant throughout a person’s life and waits for more favorable conditions (4). Given the body’s natural ability to respond quickly to infection, the majority of those infected with CMV are asymptomatic, though some may present with mild symptoms, including fever, sore throat and fatigue.
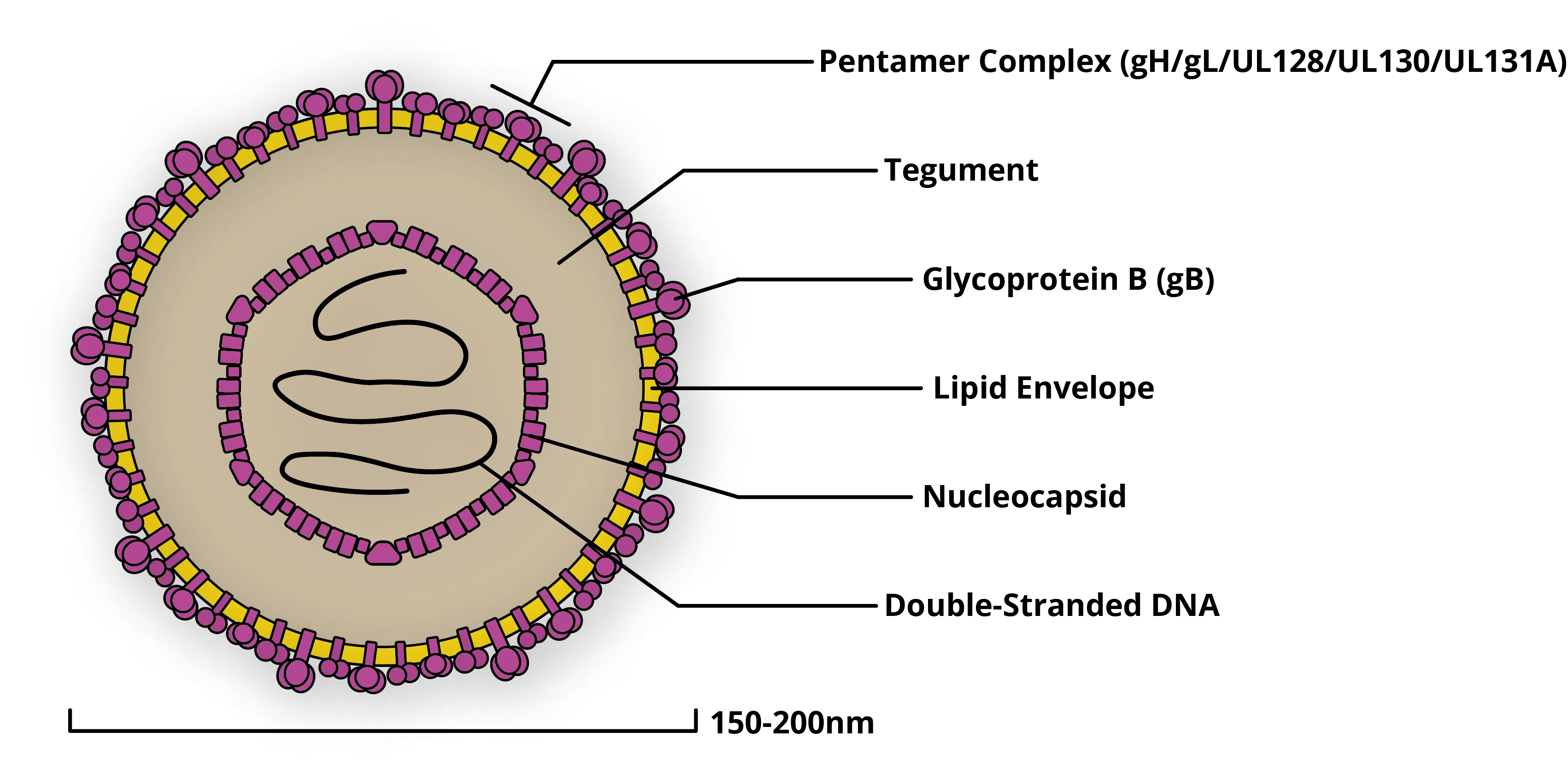
Diagram of a cytomegalovirus virion, with key proteins labelled.
The case for a CMV vaccine
Though the majority of CMV infections are often benign and of little concern, the virus poses a significant risk to those who struggle to mount an effective immune response – a problem that occurs predominantly in two groups:
The pregnant mother and fetus. If a new or reactivated CMV infection occurs during pregnancy, the virus can be transmitted to the placenta and establish a congenital infection in the developing fetus (cCMV). Here, it can wreak havoc on an infant’s developing nervous system (5), causing irreversible defects that range from hearing loss, to impaired organ growth and learning disabilities (6). The magnitude of cCMV is significant, affecting approximately double the number of children living with better-known childhood disorders, such as Down syndrome (5), and takes the top spot for infectious hearing loss in children worldwide (7).
Recipients of donated organs and stem cell transplants. CMV is also a threat to the recipients of solid organ or hematopoietic stem cell transplants during immunosuppressive therapy. These infections can occur due to the presence of CMV in the transplanted organ, viral reactivation in the recipient’s tissue, or primary infection in seronegative recipients. Transplant-acquired infections can cause conditions, such as hepatitis, pneumonia and viremia (1) – all of which increase the likelihood of transplant rejection and graft-versus-host disease. The incidence of CMV infection among transplant recipients is remarkably common, with 20–70 percent of recipients acquiring an infection in the first year post-transplant (1), making it one of the most common complications affecting the survival of transplant recipients worldwide (8).
Despite its significant burden, no effective countermeasures are currently in place to protect those at risk, with only basic screening or behavioral measures, such as hand hygiene, available (7). And while antiviral therapies have been invaluable, they are limited by toxicity and the continual emergence of viral resistance.
Much like the rubella vaccine, a novel vaccine for CMV could protect mothers and their developing infants from CMV. Similarly, a vaccine for transplant recipients would avoid the need for expensive antiviral treatments that are limited in efficacy and duration, drastically improving patient survival and procedure success rates.
No licensed vaccines are currently available, but researchers haven’t been sitting idle; the development of CMV vaccines has been ongoing for nearly 50 years (9). Major impediments to the development of a suitable vaccine have likely been down to poor general awareness of the virus and its immunological complexity. However, the acknowledgement of the clinical significance of CMV in recent years has stimulated a surge in funding and research interest.
The Current Pipeline
Attenuated vaccines
Attenuated vaccines are created by reducing the virulence of a pathogen, while still keeping them viable. They have the potential to be one of the most effective ways of vaccinating against CMV, as they can mimic a natural infection (10). The 1990s saw the development of the first attenuated vaccines – a new generation of so-called transgenic disabled infectious single cycle (DISC) strains (see Figure 2) (11). These are live viruses with selective gene deletions in their membrane proteins that allowed them to assemble after their first infection cycle, but which were unable to infect successive cells (12). By expressing only a limited subset of the viral proteome, DISCs can be propagated under controlled conditions that allow for their production in vitro, but cannot replicate in vivo, preventing latent infection (13).
The current DISC frontrunner is Merck’s V160 strain, which includes rapamycin-binding protein (RBP) insertion. In vivo, RBPs are rapidly degraded to prevent viral replication. DISC strains such as these have effectively mitigated the concerns of viral replication and latency, and could, in theory, elicit the full repertoire of antibody and T-cell responses of a natural infection. However, considering that natural CMV infections can fail to provide the immunity required to prevent a secondary infection, it remains unclear if these vaccines will offer sufficient protection. Merck’s recent phase I trials have shown good tolerance (14) and a phase II study of 2,100 women, aged 16–35 is now underway, with results expected in 2021 (15).
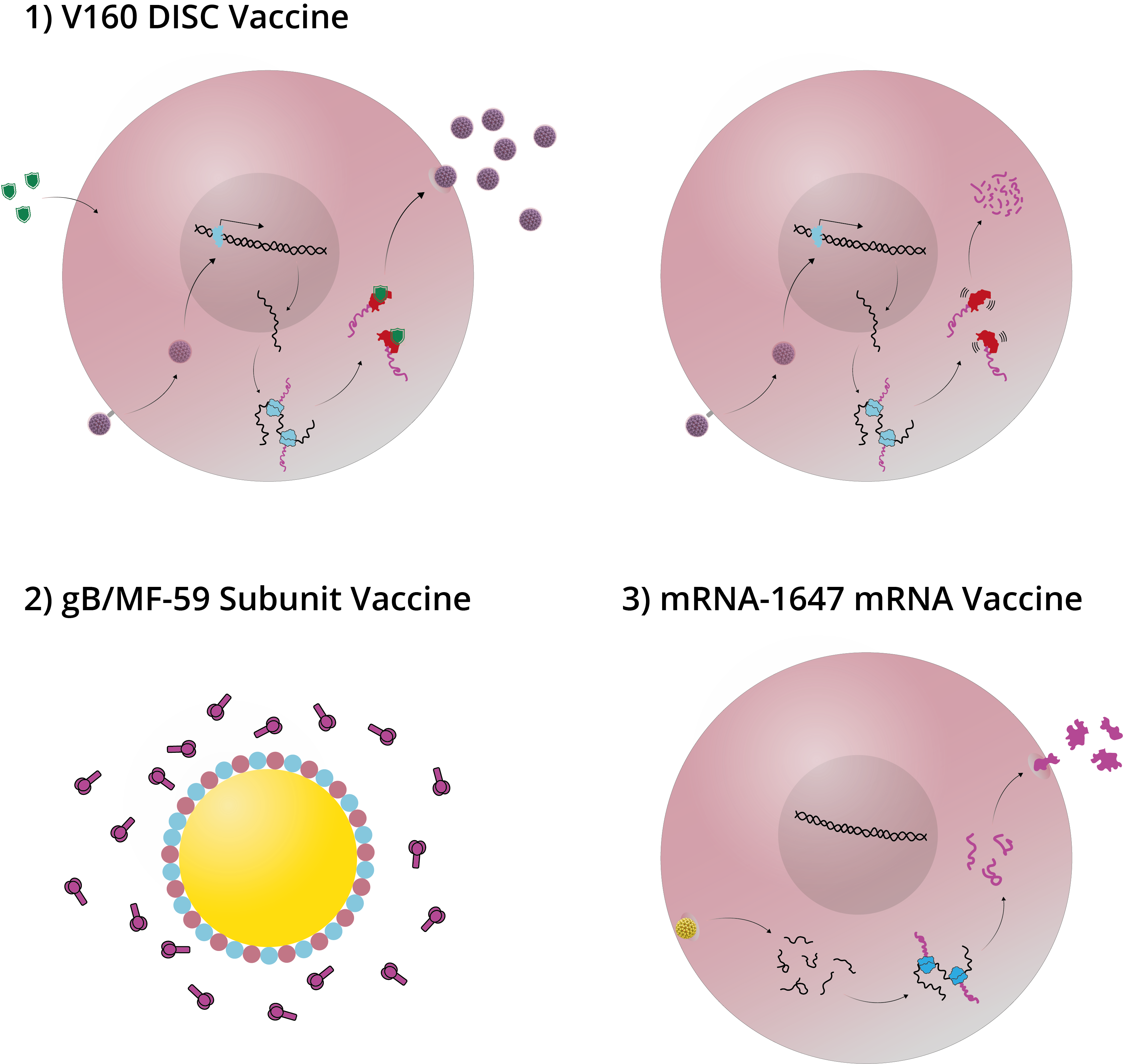
Diagram illustrating DISC, subunit and mRNA vaccines. 1) In vitro (left), Shield-1 ligand is added to cell culture alongside V160 formulation. This stabilizes the replication complex and allows the virus to proliferate; in vivo (right), no Shield-1 ligand is present, which causes destabilization of the replication complex and prevents viral assembly. 2) The adjuvant MF59 is comprised of squalene, surrounded by surfactants and formulated with subunit antigens. 3) A lipid nanoparticle fuses with cell membranes to release 6 mRNAs into the target cell cytoplasm. The mRNAs are translated by host cell machinery to produce antigens that can be detected by the immune system.
Subunit vaccines
Subunit vaccines (see Figure 2) are created using fragments of pathogens (typically surface proteins are used for their development) and are used to trigger an immune response and stimulate acquired immunity against the pathogens from which they are derived. The protein responsible for mediating CMV’s entry to human cells and the dominant target of the body’s humoral response is glycoprotein B (gB) – a homotrimeric envelope protein that has seen considerable development as an antigen in subunit vaccines. Formulated with Novartis’ proprietary MF59 adjuvant, gB has shown some promising early results by a range of vaccine developers and has arguably advanced the furthest to date in clinical trials (16).
In a 2007–2010 phase II trial of postpartum women, Sanofi Pasteur’s gB/MF59 formulation demonstrated 50 percent efficacy against primary CMV infection in seronegative women vaccinated within one year of giving birth, with acceptable adverse events (17). This landmark study was the first to demonstrate efficacy in preventing primary CMV infection, and was followed three months later by a phase II in transplant recipients that showed significantly reduced viremia and length of antiviral therapy required (18).
A glance over the literature makes it clear that no major breakthroughs with gB subunit vaccines have taken place since. A likely reason for this was the realization that CMV’s pentamer complex is also a key target for neutralizing antibodies and would be required in formulations (19). Combined with the fact that gB has reached only 50 percent efficacy, and that the immune responses to gB and pentamer stimulate protection in different cell types, it is likely that an effective vaccine will need to consist of both proteins (20).
RNA vaccines
A recent entrant to the CMV race is Moderna, which has been developing novel messenger RNA technologies since their founding in 2010. Unlike protein-based vaccines, Moderna’s mRNA-1647 formulation uses mRNA to instruct the host’s cells to produce antigens on-site, making manufacture and administration far simpler (21). The formulation comprises a lipid nanoparticle, that contains six separate mRNAs, collectively encoding five of the pentamer complex subunits and gB.
Earlier this year, Moderna released some promising phase I trial data (22). Results showed good toleration and no severe adverse events after a three-dose schedule, and boosting of neutralizing antibody titers in both seronegative and seropositive participants; 10-fold and 20–40-fold, respectively (23). Moderna are now taking their vaccine forward, making it the first mRNA vaccine for an infectious disease to enter a phase II clinical trial. Moreover, plans are already in place for a phase III in 2021, which will evaluate efficacy in up to 8,000 women of childbearing age.
Though attenuated, subunit and RNA vaccines are demonstrating varying levels of success in the clinic, there are a wide range of other vaccine strategies beyond the scope of this article, including peptide, DNA and vectored vaccines, all of which present viable options for tackling CMV.
It’s clear that the industry has made significant advances in our understanding of CMV in recent years, with a range of emerging technologies that could soon see a breakthrough. However, to develop an effective vaccine that can prevent both in utero and transplant-associated infections, some key challenges will need to be addressed.
A slightly disconcerting consideration is that we still don’t fully understand how protective immunity is conferred by natural infection with CMV (13). Though this must have made vaccine design feel like a shot in the dark for researchers in the past, emerging data is now starting to paint a more detailed picture. A vaccine will need to stimulate both the humoral and cell-mediated immune responses, as anti-CMV antibodies have been shown to prevent transmission and minimize clinical manifestations, while T-cells are likely involved in suppressing viral replication and prevent reactivation of infection (2, 23). Further research is necessary to provide a clearer picture that defines the correlates of protection.
Immunization is also challenged by the fact that natural infection with CMV is only partially protective and does not prevent placental transmission of the virus (24, 25). And that means vaccine candidates will need to provide protection exceeding that of natural infection (8), and may make the prospects for traditional vaccines somewhat limited (26).
An ongoing challenge in biologic manufacturing has been the need to maintain homogenous cell lines that can be scaled to yield vaccines of a high enough purity for use in humans. mRNA technologies, such as Moderna’s, are entirely cell-free and avoid the need for complex manufacturing processes, which would make these strategies much more practical for real-world use. However, it has yet to be seen whether mRNA vaccines are able to consistently elicit potent and long-lasting immune responses to CMV.
Another serious consideration: how to practically evaluate efficacy in trials. cCMV is fairly rare at the population level (about 1 in 150 pregnancies) and occurs in mostly vulnerable patient populations. In practice, this requires large patient samples in resource-limited populations, which may deter more risk-averse developers. Likewise, determining practical endpoints can be a headache when you consider that viral transmission, prevention of maternal infection, blocking of cross-placental transmission, reduction in congenital infection in infants, transplant survivability, and reduction in antiviral treatment are all clinically relevant (27).
Despite these challenges, the industry remains optimistic. Successful phase III trials from vaccine front-runners, such as V160 and mRNA-1647, could clear the path to market in the coming years. And though the road towards a CMV vaccine has been long and bumpy, encouraging results from a wide scope of vaccine types suggests that we’re getting closer to a future where CMV poses less of a risk to those most vulnerable to infection.
To support ongoing CMV research, The Native Antigen Company provides a range of antigens and antibodies for vaccine and diagnostic development:
Antigens
The Native Antigen Company have developed a range of CMV antigens, including preparations from native virus and highly purified recombinant proteins, expressed in our proprietary VirtuE mammalian cell expression system. Our recombinant antigens include the two key vaccine candidates, glycoprotein B, with mouse and human Fc tags, and CMV pentamer protein, for which The Native Antigen Company is renowned. We also offer purified whole CMV antigen and CMV cell lysate.
Antibodies
The Native Antigen Company offers a range of fully validated anti-CMV antibodies using our antigen range. This range includes CMV-specific antibodies against glycoprotein B, pentamer protein, glycoprotein H, ICP36 and phosphoproteins 28 and 65.
Our sheep anti-CMV pentamer polyclonal has been raised against mammalian-expressed gH pentameric complex, and has been shown to react with each of the components of the pentamer in Western blotting analysis. Our mouse anti-glycoprotein B monoclonal recognises and binds a neutralising epitope on CMV glycoprotein B and has also shown to be effective at binding in direct ELISA.
References
- Ross, A. et al. “Mixed Infection and Strain Diversity in Congenital Cytomegalovirus Infection”, The Journal of Infectious Diseases, 204(7), 1003-1007 (2011).
- Cui, X. and Snapper, C. M. “Development of novel vaccines against human cytomegalovirus”, Human Vaccines & Immunotherapeutics, 15(11), 2673-2683 (2019).
- Plotkin, S. A. and Boppana, S, B. “Vaccination against the human cytomegalovirus”, Vaccine, 37(50), 7437-7442 (2019).
- Fields, B. N., Knipe, D. M., Howley, P. M. ‘Fields Virology’, Wolters Kluwer Health, 1-3091 (2007).
- Cheeran, M. C.-J., Lokensgard, J. R., Schleiss, M. R. “Neuropathogenesis of Congenital Cytomegalovirus Infection: Disease Mechanisms and Prospects for Intervention”, Clinical Microbiology Reviews, 22(1), 99-126 (2009).
- Dollard, S. C., Grosse, S. D., Ross, D. S. “New Estimates of the Prevalence of Neurological and Sensory Sequelae and Mortality Associated With Congenital Cytomegalovirus Infection”, Reviews in Medical Virology, 17(5), 355-363 (2007).
- Boppana, S. B., Ross, S. A., Fowler, K. B. “Congenital Cytomegalovirus Infection: Clinical Outcome”, Clinical Infectious Diseases, 57, S178-S181 (2013).
- Diamond, D. J. et al. “A fifty-year odyssey: Prospects for a cytomegalovirus vaccine in transplant and congenital infection”, Expert Review of Vaccines, 17(10), 889-911 (2018).
- Plotkin, S. A. “The history of vaccination against cytomegalovirus”, Medical Microbiology and Immunology, 204(3), 247-254 (2015).
- Choi, K. Y., Root, M., McGregor, A. “A Novel Non-Replication-Competent Cytomegalovirus Capsid Mutant Vaccine Strategy Is Effective in Reducing Congenital Infection”, Journal of Virology, 90(17), 7902-7919 (2016).
- Stern, H. “Live Cytomegalovirus Vaccination of Healthy Volunteers: Eight-Year Follow-Up Studies”, Birth Defects original article series, 20(1), 263-269 (1984).
- Anderholm, K. M., Bierle, C. J., Schleiss, M. R. “Cytomegalovirus Vaccines: Current Status and Future Prospects”, Drugs, 76(17), 1625-1645 (2016).
- Koelle, D. M. and Corey, L. “Recent Progress in Herpes Simplex Virus Immunobiology and Vaccine Research”, Clinical Microbiology Reviews, 16(1), 96-113 (2003).
- Reddehase, M. J. “Cytomegaloviruses: From Molecular Pathogenesis to Intervention”, Caister Academic Price, 1-464 (2013).
- Fu, T.-M., An, Z., Wang, D. “Progress on Pursuit of Human Cytomegalovirus Vaccines for Prevention of Congenital Infection and Disease”, Vaccine, 32(22), 2525-2533 (2014).
- Safety and Immunogenicity of the Human Cytomegalovirus (CMV) Vaccine (V160) in Healthy Japanese Men (V160-003). Available at: https://clinicaltrials.gov/ct2/show/NCT03840174
- “Safety, Tolerability, and Efficacy of the Human Cytomegalovirus Vaccine (V160) in Healthy Women 16 to 35 Years of Age (V160-002)”. Available at: https://clinicaltrials.gov/ct2/show/NCT03486834
- “Recombinant CMV gB Vaccine in Postpartum Women”. Available at: https://clinicaltrials.gov/ct2/show/NCT00125502
- “CMV Glycoprotein B Vaccine in Allograft Recipients”. Available at: https://clinicaltrials.gov/ct2/show/NCT00299260
- Wussow, F. et al. “Human Cytomegalovirus Vaccine Based on the Envelope gH/gL Pentamer Complex”, PLoS Pathogens, 10(11), (2014).
- Rieder, F. and Steininger, C. “Cytomegalovirus vaccine: phase II clinical trial results”, Clinical Microbiology and Infection, 20(Supplement 5), 95-102 (2014).
- Zhang, C. et al. “Advances in mRNA Vaccines for Infectious Diseases”, Frontiers in Immunology, 10(594), (2019).
- “Moderna Announces Additional Positive Phase 1 Data from Cytomegalovirus (CMV) Vaccine (mRNA-1647) and First Participant Dosed in Phase 2 Study”. Available at: https://investors.modernatx.com/news-releases/news-release-details/moderna-announces-additional-positive-phase-1-data
- Sylwester, A. W. et al. “Broadly targeted human cytomegalovirus-specific CD4+and CD8+ T cells dominate the memory compartments of exposed subjects”, Journal of Experimental Medicine, 202(5), 673-685, (2005).
- Rosa, C. L. and Diamond, D. J. “The immune response to human CMV”, Future Medicine, 7(3), 279-293, (2012).
- Bialas, K. M. and Permar, S. R. “The March towards a Vaccine for Congenital CMV: Rationale and Models”, PLoS Pathogens, 12(2), (2016).
- Pass, R. F. et al. “Vaccine Prevention of Maternal Cytomegalovirus Infection”, New England Journal of Medicine, 360(12), 1191-1199, (2009).
- Nelson, C. S., Betsy, C. H., Permar, S. R. (2018). “A new era in cytomegalovirus vaccinology: considerations for rational design of next-generation vaccines to prevent congenital cytomegalovirus infection”, NPJ Vaccines, 3(38), (2018).